Unique transportable instruments
QuantumFrontiers has enhanced the accessibility and robustness of quantum metrology, making precision measurements more widely available for critical applications in geodesy and environmental sensing. Transportable optical clocks have been pioneered by QuantumFrontiers researchers, now achieving unprecedented uncertainty levels (< 10–17). They have been used, e.g. in a recent campaign to determine the height difference between PTB Braunschweig and Max Planck Institute of Quantum Optics in Garching, separated by 940 km of fibre, with a record 24 cm uncertainty [3]. Complementing clocks, QuantumFrontiers researchers developed and optimised the unique transportable quantum gravimeter QG-1, based on an atom-chip BEC source for high-accuracy observations. QG-1 operated continuously for over a week and achieved accuracy of less than 10 nm/s2 [4, 5].
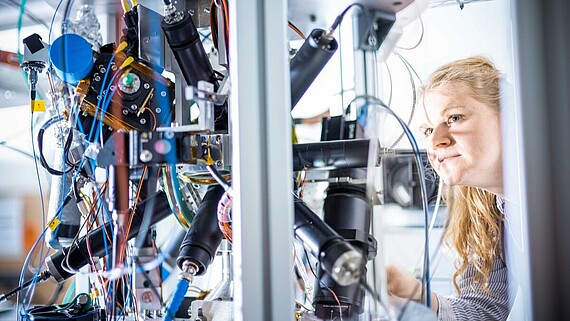
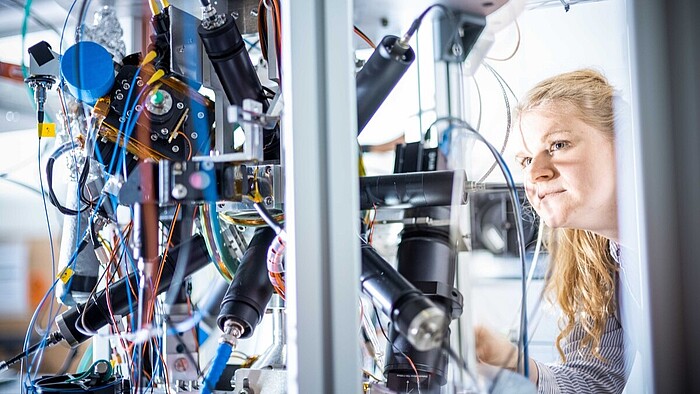
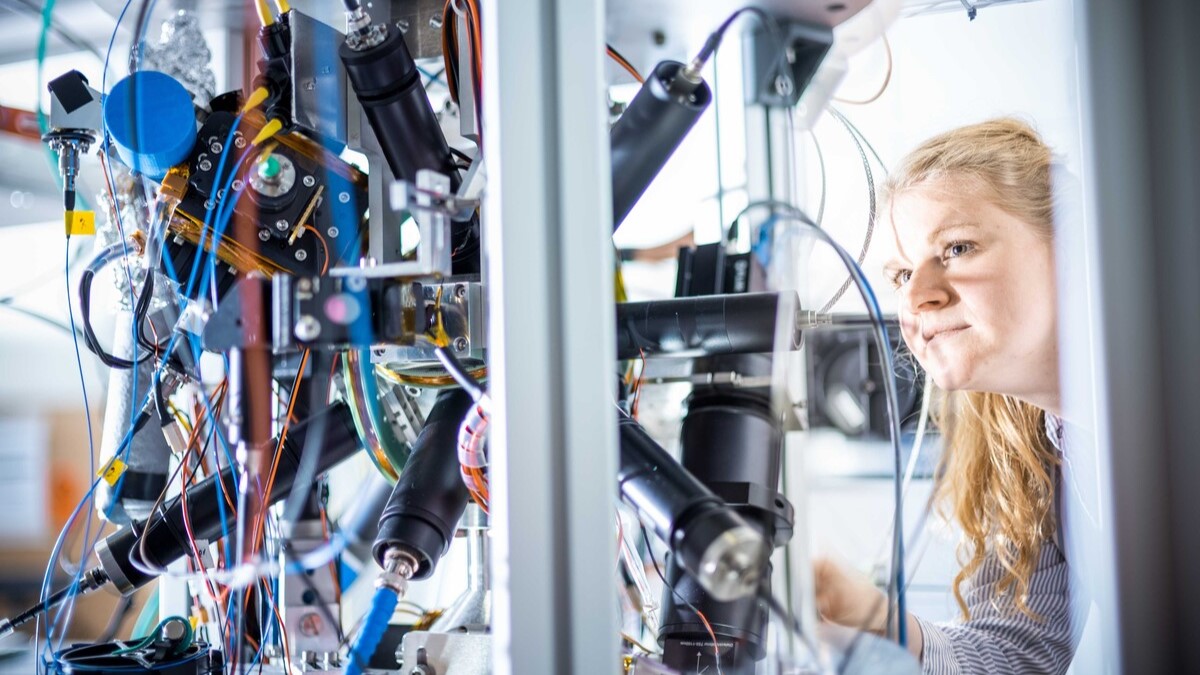
Towards ubiqitous sensing
In interferometric inter-spacecraft ranging, QuantumFrontiers leveraged synergies with the LISA mission and developed an optical interferometer for the Gravity Recovery and Climate Experiment Follow-On (GRACE-FO) satellite mission [6]. They lead the instrument development [7] for the upcoming Mass-Change and Geosciences International Constellation (MAGIC) mission. This includes novel laser frequency determination schemes [8], a new optical head for multi-degree-of-freedom inertial sensing [9], and improved test mass sensing and accelerometry solutions [10]. GRACE-FO has been used to study the global hydrological cycle [11].
A notable extension of this collaborative success story towards environmental metrology is the expertise of J. Daniel Prades, who joined TU Braunschweig in 2024 as Alexander-von-Humboldt professor. He advances our activities in ubiquitous metrology by developing innovative sensor concepts, holding performance records in zero-power chemical sensors, miniaturised nano-heaters and microLEDs optimised for highly-localised irradiation [12–14].
This article is part of a series on QuantumFrontiers success stories
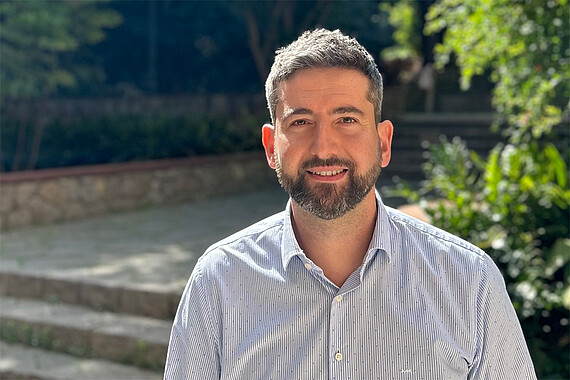
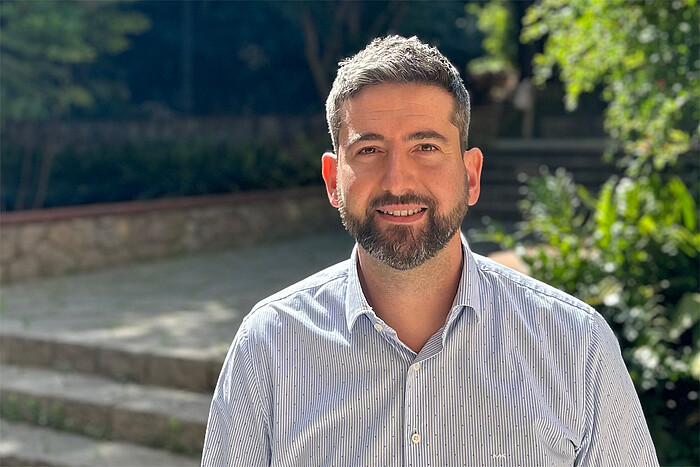
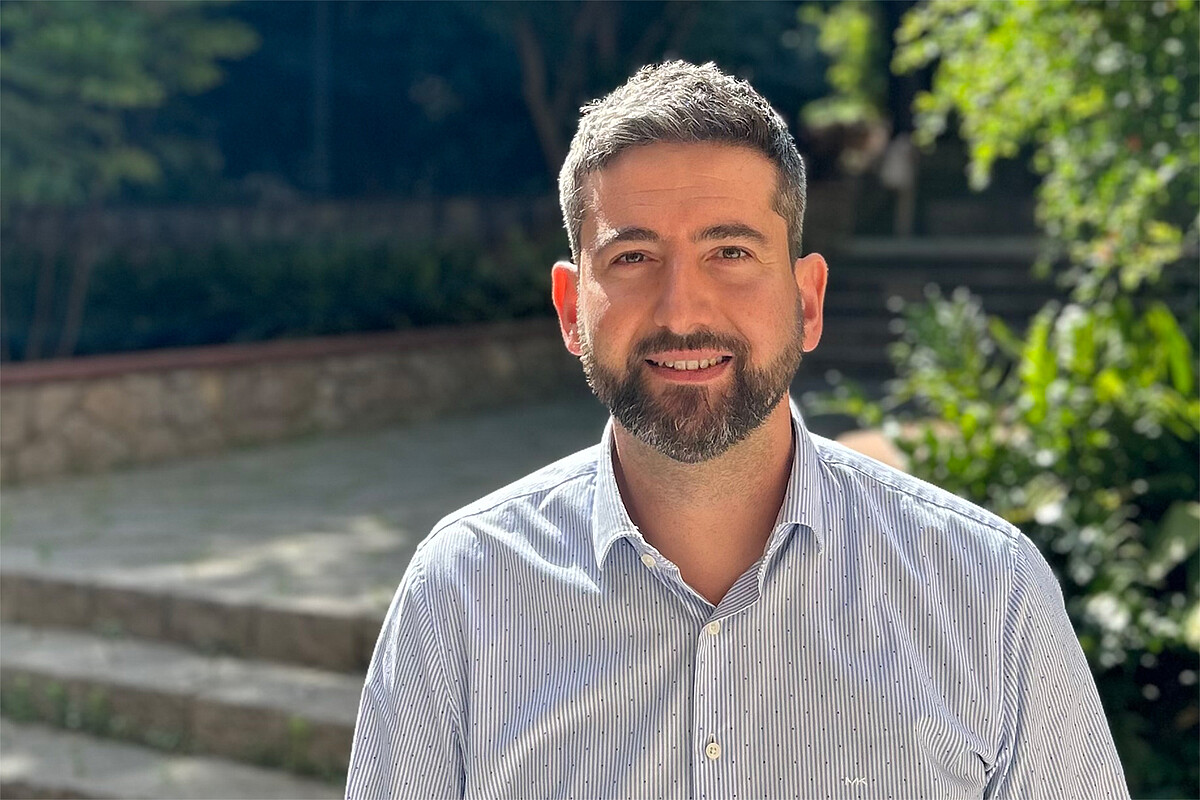
Publications
-
Bibliography
[1] A. Vincent, J. Müller. Detection of time variable gravity signals using terrestrial clock networks.
Advances in Space Research 73, 3312–3320. doi:10.1016/j.asr.2023.07.058 (2024).[2] A. Shabanloui, H. Wu, J. Müller. Estimation of Temporal Variations in the Earth’s Gravity Field Using Novel Optical Clocks Onboard of Low Earth Orbiters.
In: J.T. Freymueller, L. Sánchez (eds.) Gravity, Positioning and Reference Frames. REFAG 2022. IAG Symposia, vol 156. Springer, Cham. doi:10 . 1007/1345 _ 2023 _ 220 (2023).[3] J. Grotti, I. Nosske, et int, C. Lisdat. Long-distance chronometric leveling with a portable optical clock.
Physical Review Applied 21. doi:10.1103/physrevapplied.21.l061001 (2024).[4] C. Deppner, W. Herr, et int, E. M. Rasel. Collective-Mode Enhanced Matter-Wave Optics.
Physical Review Letters 127. doi:10.1103/physrevlett.127.100401 (2021).[5] J. Kirsten-Siemß, F. Fitzek, et int, K. Hammerer. Large-Momentum-Transfer Atom Interferometers with μ rad-Accuracy Using Bragg Diffraction.
Physical Review Letters 131. doi:10.1103/PhysRevLett.131. 033602 (2023).[6] K. Abich, A. Abramovici, et int, M. Zimmermann. In-Orbit Performance of the GRACE Follow-on Laser Ranging Interferometer.
Physical Review Letters 123. doi:10.1103/physrevlett.123.031101 (2019).[7] K. Nicklaus, K. Voss, et int, J. J. E. Delgado. Towards NGGM: Laser Tracking Instrument for the Next Generation of Gravity Missions.
Remote Sensing 14, 4089. doi:10.3390/rs14164089 (2022).[8] M. Misfeldt, V. Müller, et int, G. Heinzel. Scale Factor Determination for the GRACE Follow-On Laser Ranging Interferometer Including Thermal Coupling.
Remote Sensing 15. doi:10.3390/rs15030570 (2023).[9] V. Huarcaya, M. Dovale Álvarez, et int, G. Heinzel. Single-Element Dual-Interferometer for Precision Inertial Sensing: Sub-Picometer Structural Stability and Performance as a Reference for Laser Frequency Stabilization.
Sensors 23, 9758. doi:10.3390/s23249758 (2023).[10] A. Kupriyanov, A. Reis, et int, J. Müller. Benefit of enhanced electrostatic and optical accelerometry for future gravimetry missions.
Advances in Space Research 73, 3345–3362. doi:10.1016/j.asr.2023.12.067 (2024).[11] A. Klemme, T. Warneke, et int, C. Lämmerzahl. Sediment transport in South Asian rivers high enough to impact satellite gravimetry.
Hydrology and Earth System Sciences 28, 1527–1538. doi:10.5194/hess-28-1527-2024 (2024).[12] M. W. G. Hoffmann, L. Mayrhofer, et int, J. D. Prades. A Highly Selective and Self-Powered Gas Sensor Via Organic Surface Functionalization of p-Si/n-ZnO Diodes.
Advanced Materials 26, 8017–8022. doi:10.1002/ adma.201403073 (2014).[13] C. Fàbrega, O. Casals, F. Hernández-Ramírez, J. Prades. A review on efficient self-heating in nanowire sensors: Prospects for very-low power devices.
Sensors and Actuators B: Chemical 256, 797–811. doi:10.1016/j.snb.2017.10.003 (2018).[14] N. Markiewicz, O. Casals, et int, J. D. Prades. Micro light plates for low-power photoactivated (gas) sensors.
Applied Physics Letters 114. doi:10.1063/1.5078497 (2019)