Weltbeste Quetschtlichtquellen
Die Entwicklung der weltbesten Quellen für gequetschtes Licht in Hannover war von zentraler Bedeutung, um die außerordentliche Empfindlichkeit aktueller Gravitationswellendetektoren zu erreichen. GEO600 war der erste Detektor, der routinemäßig nicht-klassisches Licht verwendete, und die Forschenden von QuantumFrontiers halten den Rekord für den höchsten Grad an Licht-Quetschung (6 dB) in einem vollwertigen Gravitationswellendetektor [1]. Sie haben die Quelle für gequetschtes Licht für Advanced Virgo entwickelt und installiert [2, 3] und so die astrophysikalische Reichweite des Detektors vergrößert [4]. Zudem haben sie einen entscheidenden Beitrag zum Hochleistungslasersystem für aLIGO geleistet [5, 6]. Erst die dadurch erhöhte Empfindlichkeit der Detektoren hat den ersten direkten Nachweis von Gravitationswellen ermöglicht und den gemeinsamen Betrieb dieser Detektoren als Teil des globalen Detektornetzwerks erlaubt.
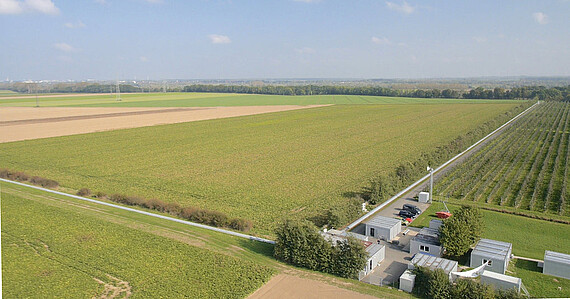
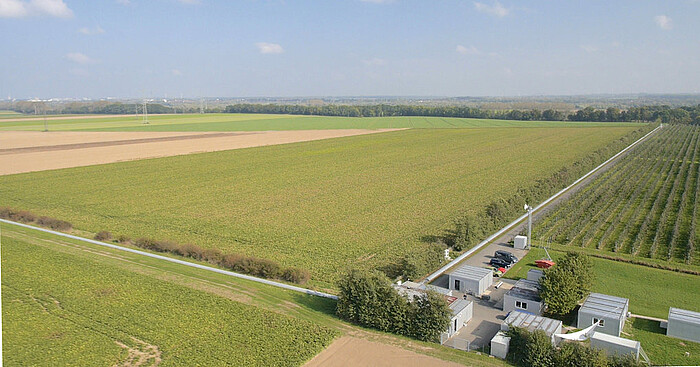
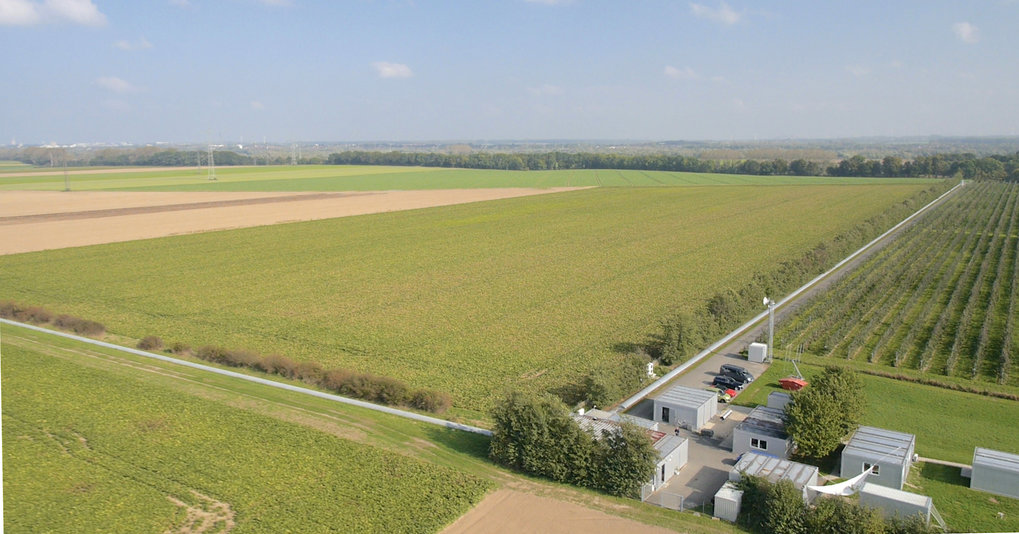
Um künftige Gravitationswellendetektoren mit noch höherer Empfindlichkeit und damit bahnbrechende Entdeckungen in der Astrophysik und der Grundlagenphysik zu ermöglichen, haben Forschende von QuantumFrontiers frequenzabhängig gequetschtes Licht ohne externe optische Resonatoren erzeugt [7], eine Rauschreduzierung von über 13 dB bei der Laserwellenlänge von 1550 nm erreicht [8] und das Quetschen in transversalen Moden höherer Ordnung nachgewiesen [9]. Auch bei der kohärenten Verringerung des Druckrauschens von Quantenstrahlung wurden bedeutende Fortschritte erzielt, und die erste Integration optischer und optomechanischer Teilsysteme steht kurz bevor [10].
Forschung für die nächste Generation von Gravitationswellendetektoren
Der AEI 10 m Prototyp, eine Großforschungsanlage mit einem Fabry-Pérot-Michelson-Interferometer, das am Standard-Quantenlimit (SQL) arbeitet, wird die Infrastruktur für die Erprobung dieser neuartigen Quantentechnologien bereitstellen. Für die äußerst erfolgreiche LISA Pathfinder-Mission [11] entwickelten die Forschenden von QuantumFrontiers optische Sensoren für die Auslesung der Massenverschiebung [12], untersuchten die interferometrische Datenerfassung im Labor und überwachten den täglichen Betrieb. Sie analysierten optische Rauschquellen [13] und das Rauschen, das aus der Kopplung von Kipp- und Längenänderung resultiert [14]. Ihre Beiträge waren von entscheidender Bedeutung, da sie die Funktionsweise kritischer Hardware demonstriert und wesentliche wissenschaftliche Erkenntnisse geliefert haben.
Dieser Artikel ist Teil einer Serie über die Erfolge von QuantumFrontiers
Dank dieser Leistungen ist die LISA-Mission auf dem besten Weg, Mitte der 2030er Jahre zu starten, wobei QuantumFrontiers-Wissenschaftlerinnen und -Wissenschaftler für das interferometrische Detektionssystem, die Entwicklung des Phasenmeters und die Rauschleistungsstudien verantwortlich sind. Über die LISA-Mission hinaus werden Konzepte zur Miniaturisierung optischer Sensoren untersucht [15], eine Torsionswaage als Kraftprüfstand entwickelt [16] und Anstrengungen zur Reduzierung von optischen Rauschquellen unternommen [17].
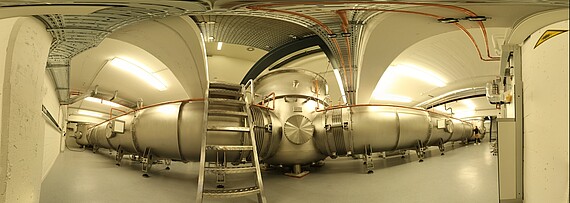
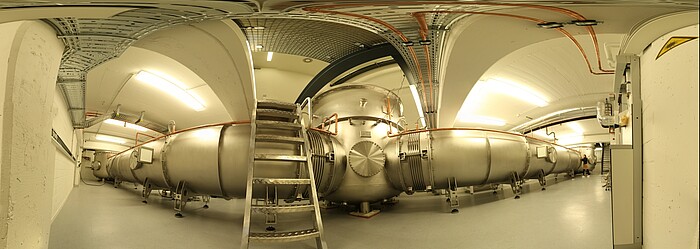
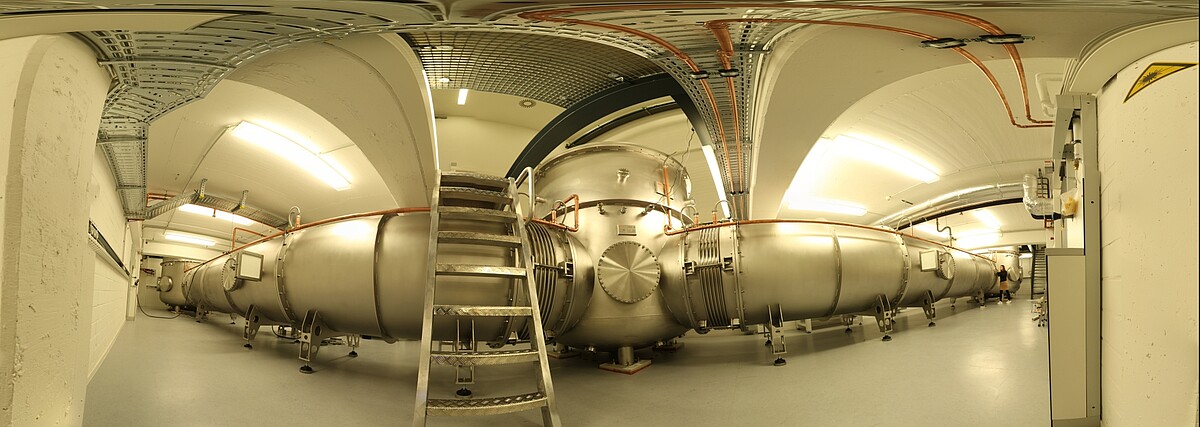
Schließlich spielen die Forschenden von QuantumFrontiers eine Schlüsselrolle bei Gravitationswellendetektoren im Infraschallbereich unter Verwendung der Materiewellen-Interferometrie. Im Rahmen der terrestrischen VLBAI-Kollaboration wurden verschiedene Detektorkonzepte mit Partnern in den USA (MAGIS) und China (ZAIGA) erprobt [18]. Prototypen im 10-Meter-Maßstab sind einsatzbereit, darunter die VLBAI-Anlage in Hannover. Im Rahmen des Europäischen Labors für Gravitations- und Atom-Interferometrie-Forschung (ELGAR) bereiten die Forschenden von QuantumFrontiers Studien für eine europaweite Infrastruktur für eine 3D-Infraschall-Gravitationswellen-Antenne mit gequetschten Atomquellen vor.
Publikationen
-
Literaturliste
[1] J. Lough, E. Schreiber, et int, K. Danzmann. First Demonstration of 6 dB Quantum Noise Reduction in a Kilometer Scale Gravitational Wave Observatory.
Physical Review Letters 126. doi:10.1103/physrevlett.126. 041102 (2021).[2] F. Acernese, M. Agathos, et int, K. Danzmann. Increasing the Astrophysical Reach of the Advanced Virgo Detector via the Application of Squeezed Vacuum States of Light.
Physical Review Letters 123. doi:10.1103/physrevlett.123.231108 (2019).[3] M. Mehmet, H. Vahlbruch. The Squeezed Light Source for the Advanced Virgo Detector in the Observation Run O3.
Galaxies 8, 79. doi:10.3390/galaxies8040079 (2020).[4] F. Acernese, M. Agathos, et int, K. Danzmann. Frequency-Dependent Squeezed Vacuum Source for the Advanced Virgo Gravitational-Wave Detector.
Phys. Rev. Lett. 131, 041403. doi:10.1103/PhysRevLett.131.041403 (2023).[5] F. Wellmann, N. Bode, et int, D. Kracht. Low noise 400 W coherently combined single frequency laser beam for next generation gravitational wave detectors.
Optics Express 29, 10140. doi:10.1364/oe.420350 (2021).[6] F. Wellmann, M. Steinke, et int, D. Kracht. Performance study of a high-power single-frequency fiber amplifier architecture for gravitational wave detectors.
Appl. Opt. 59, 7945. doi:10.1364/ao.401048 (2020).[7] J. Junker, D. Wilken, et int, M. Heurs. Frequency-Dependent Squeezing from a Detuned Squeezer.
Physical Review Letters 129. doi:10.1103/physrevlett.129.033602 (2022).[8] F. Meylahn, B. Willke, H. Vahlbruch. Squeezed States of Light for Future Gravitational Wave Detectors at a Wavelength of 1550 nm.
Phys. Rev. Lett. 129, 121103. doi:10.1103/PhysRevLett.129.121103 (2022).[9] J. Heinze, B. Willke, H. Vahlbruch. Observation of Squeezed States of Light in Higher-Order Hermite-Gaussian Modes with a Quantum Noise Reduction of up to 10 dB.
Phys. Rev. Lett. 128, 083606. doi:10.1103/PhysRevLett.128.083606 (2022).
[10] J. Schweer, D. Steinmeyer, K. Hammerer, M. Heurs. All-optical coherent quantum-noise cancellation in cas-caded optomechanical systems.
Physical Review A 106. doi:10.1103/physreva.106.033520 (2022).
[11] M. Armano, H. Audley, et int, P. Zweifel. Sub-Femto-g Free Fall for Space-Based Gravitational Wave Observatories: LISA Pathfinder Results.
Physical Review Letters 116. doi:10.1103/physrevlett.116.231101 (2016).
[12] M. Armano, H. Audley, et int, P. Zweifel. Sensor Noise in LISA Pathfinder: In-Flight Performance of the Optical Test Mass Readout.
Physical Review Letters 126. doi:10.1103/physrevlett.126.131103 (2021).
[13] M. Armano, H. Audley, et int, P. Zweifel. Sensor noise in LISA Pathfinder: An extensive in-flight review of the angular and longitudinal interferometric measurement system.
Physical Review D 106. doi:10.1103/physrevd.106.082001 (2022).[14] M. Armano, H. Audley, et int, P. Zweifel. Tilt-to-length coupling in LISA Pathfinder: A data analysis.
Physical Review D 108. doi:10.1103/physrevd.108.102003 (2023).[15] K.-S. Isleif, G. Heinzel, M. Mehmet, O. Gerberding. Compact Multifringe Interferometry with Subpicometer Precision.
Physical Review Applied 12. doi:10.1103/physrevapplied.12.034025 (2019).[16] G. Bergmann, C. Cordes, et int, M. Mehmet. A torsion balance as a weak-force testbed for novel optical inertial sensors.
Classical and Quantum Gravity 41, 075005. doi:10.1088/1361-6382/ad29e8 (2024).[17] M.-S. Hartig, S. Schuster, G. Heinzel, G. Wanner. Non-geometric tilt-to-length coupling in precision interferometry: mechanisms and analytical descriptions.
J. of Optics 25. doi:10.1088/2040-8986/acc3ac (2023).[18] S. Abend, B. Allard, et int, E. Zupanič. Terrestrial Very-Long-Baseline Atom Interferometry: Workshop Summary.
arXiv: 2310.08183 [hep-ex] (2023).